A
lever is a mechanical part that has arms and a fixed pivot used to transmit
torque. The cross section of the arm is usually a rectangular shape which makes it stiff against bending. However, its stiffness may become lower if the loading point is not on the right place. We're going to see the effect of torsion on the lever with the improvement idea.
The following picture is the components of a general
cam and lever system. The lever has the
hub in the middle. It's where bearings are placed inside and it's the fixed pivoting point on a
lever shaft (not shown). There are 2 arms to the left and the right. They're both welded to the hub and rotate together. The right arm is connected to a
cam follower that rolls over
cam surface. When the cam rotates the lever will swing up and down since there will be spring forces pushing the cam follower to keep contact with the cam surface all the time. The left arm has another point to connect to other machine parts, which usually is a
pull rod. It will rotate at the same angle (degrees) with the right arm, but the distance (mm) may be different depending on a
lever ratio.
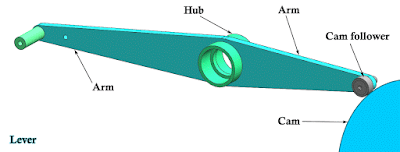 |
Components of cam & lever system |
The
pull rod connecting point on the left in this example is not good since it has a long distance from the arm and the arm will not only be subjected to bending but also torsion. The following is the lever in the machine.
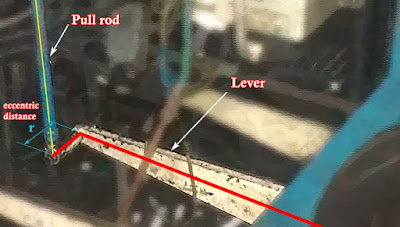 |
Lever with eccentric pull rod connecting point |
The eccentric distance "r" usually comes from space limitation on the lever shaft. There may be some other machine parts blocking the lever and it can't move any further. The hub stays at the same location and the lever is just straight from the hub. Because of this, there will be a gap between the lever and the pull rod. That's why the designer add that small connection post at the end.
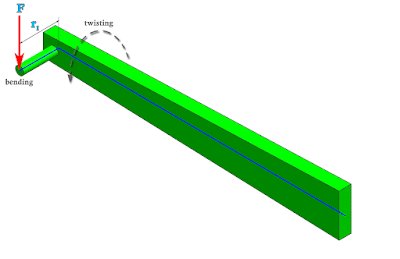 |
Load from the pull rod |
The small post will be subjected to bending and the lever arm will also subjected to bending as well as torsion. If the distance "r
1" is much less then it could improve the stiffness. Here is the finite element analysis result of this lever.
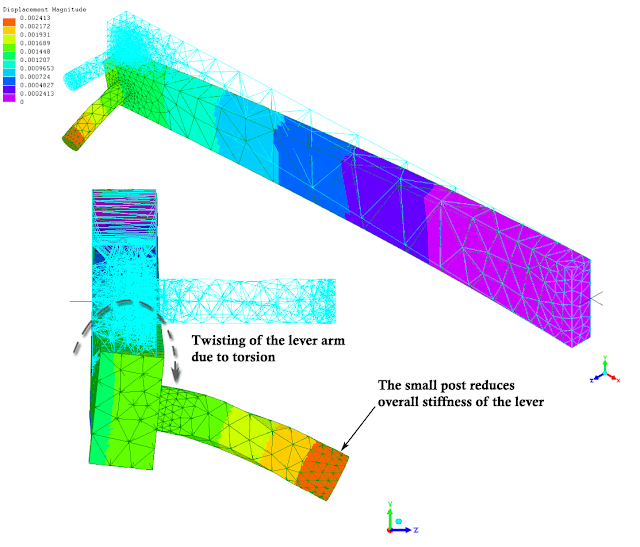 |
Finite element analysis - displacement of the lever under eccentric load |
We can see from the finite element analysis result that the lever bends down due to the load and it also twists to the right because of the eccentric load. However, the small post that connects to the pull rod reduces the overall stiffness since it also bends down.
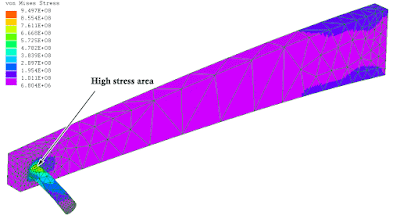 |
von Mises stress of the lever under torsional load |
The highest
von Mises stress is at the weakest point on the small post as shown in the picture.
To reduce the overall displacement, the overall stiffness will improve. We can change some designs to eliminate the torsion away from the lever arm and reduce the length of the post. Let's see how it's done in the
next post.